Look Ahead: An Exciting Future for Emerging CT Platforms
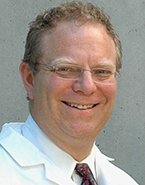
Over the past 30 years, I have often heard CT branded as a mature technology, characterizing opportunities for its advancement as incremental at best. Just when I begin to wonder if they might be right, I am invariably delighted by unforeseen technological advances that transform the clinical application of CT.
CT was considered a mature technology through the mid to late 1980s, when Willi Kalendar introduced the world to spiral CT, eliminating the scourge of respiratory misregistration by enabling imaging of entire organs during a single breath-hold and capturing the arterial phase of intravenous iodine injections for CT angiography.
For a few years, CT development seemed to slow again until 1998 when multidetector-row CT emerged, allowing acquisition of isotropic imaging volumes that expanded image interpretation beyond primary transverse reconstructions to volumetric visualization and analysis. Novel applications emerged into mainstream practice expanding CT into new clinical roles for lung cancer screening, coronary artery disease diagnosis, and characterization of cerebral ischemia.
After the row races slowed down and radiation exposure rose as a concern, iterative reconstruction and low kV imaging emerged to reduce radiation dose by as much as an order of magnitude. The demand for higher temporal resolution to freeze cardiac motion was met with faster gantry rotations, dual-source geometries, and post-processing algorithms. Finally, dual-energy CT acquisition introduced us to simulated monoenergetic reconstructions and material decomposition, albeit with caveats for those hoping to reliably create virtual unenhanced images and remove arterial calcification from arterial walls.
So now in 2019, we wonder what new CT platform advancements will appear to initiate the next wave of clinical innovations and when might we expect them? While surprises undoubtedly await us, evidence can be found like nuggets of gold glimmering from within the vast stream of medical and scientific literature. For this article, I emphasize near-term clinical innovations with only brief mention of longer-term and less certain advances.
X-Ray Source and Gantry
In the near term, major source redesign is unlikely, but we can expect continued increases in power output from smaller X-ray tubes. These advances should bring further increases in gantry rotation speed, improving temporal resolution for cardiovascular applications. While highly novel field and photo emission sources have been developed and entice us with the prospect of radical redesigns of the entire CT system, their appearance in the clinic is likely to be beyond a five-year horizon.
Photon Counting Detectors
In contrast to the source and gantry systems, we are at the cusp of a major advancement in detector technology. Over the past decade, commercially available solutions for dual-energy or multispectral CT have provided a basis for producing simulated monoenergetic images, virtual unenhanced images, metal artifact reduction and material decomposition. These advances have allowed higher sensitivity to iodinated contrast material enhancement, characterization of native tissue attenuation without a dedicated pre-contrast enhanced scan, and quantification of elements and molecules with distinct electron density and K-shell ionization energy signatures, including iodine, calcium, urate, silicone and gold nanoparticles. The value of these latter capabilities has been demonstrated for characterizing renal, pancreatic and hepatic lesions, renal calculi, bone mineralization, tophaceous gout and accumulation of silicone in lymph nodes following rupture of breast implants. Early experimentation visualizing gold nanoparticles for blood pool imaging as well as passively and actively targeted tumor imaging have been enabled using multispectral techniques.
As exciting as these advancements have been, photon-counting detectors (PCDs) are poised to greatly improve and expand these capabilities. Currently prevalent in SPECT, PET and small-bore CT systems, PCDs must be designed differently to accommodate the higher photon flux of CT systems, but progress is being made.
Energy integrating detectors intrinsic to current clinical CT systems rely on the conversion of visible light to electrical signals through a chain that flows from scintillator to photodiode with the resultant output being proportional to the total energy of the detected photons. Thus, higher energy photons contribute more to the output signal than low energy photons. In contrast, PCDs directly convert the energy of X-ray photons to electric energy. Because a scintillator is no longer needed, detector elements can be smaller and packed tighter providing a basis for scans with higher spatial resolution than currently available. Moreover, PCDs count incident photons discretely, providing a means of measuring and then binning each photon detection into one of 2–8 bins based upon its energy with the number of bins being determined by the PCD’s design.
"So now in 2019, we wonder what new CT platform advancements will appear to initiate the next wave of clinical innovations and when we might expect them.”
Geoffrey D. Rubin, MD
Clinical Implications
The potential clinical payoffs of PCDs are many. A reduction in electronic noise will provide high-quality images using lower radiation exposure and in larger patients. The relatively greater influence of low energy photons favors the photoelectric effect and thus greater iodine sensitivity, particularly when scanning larger patients with higher X-ray tube potentials. On the other end of the energy scale, weighting high-energy bins reduces beam-hardening and metal artifacts. Higher spatial resolution could improve a variety of applications particularly in assessing pulmonary, osseous and cardiovascular structures. This includes metallic implants such as stents and stent-grafts as well as characterization and measurement of atherosclerotic plaque and its influence on luminal dimensions.
Finally, multi-spectral applications will be acquired with complete spatial and temporal registration using a single X-ray source, tube potential and detector layer, eliminating many current limitations.
User-defined energy thresholding will enhance flexibility, allow greater selection and post-scan filtering of iodine and calcium. The application will also optimize discrimination of novel contrast agents, allowing new applications based upon the simultaneous discrimination of multiple contrast agents.
Phase-Contrast CT
One intriguing advance that offers a fundamentally different contrast mechanism from current attenuation-based clinical systems bears mention. Phase-contrast CT images the deflection of photons that undergo Compton scattering and produces novel tissue contrast that accentuates tissue interfaces both at macroscopic and microscopic scales. It is also capable of quantifying electron density as a basis for material classification.
Although its clinical adoption in a whole-body system is likely to be more than five years off, we may see an earlier translation to small systems dedicated to breast or extremity imaging.
Data Science Inside
For most of CT’s evolution, detectors, tubes and gantries have grabbed the headlines, while software served as supporting cast to the hardware. However, data collection subsystems and associated software refinements have played a largely unsung role in advancing CT technology. Software refinements contribute to the improved effectiveness of dual-energy methods seen in recent years. However, these improvements are expected to pale with the growing incorporation of machine learning (ML) techniques for the control and analysis of CT data.
While health technology news bombards the public with claims of algorithms besting experts at diagnostic and predictive tasks, a quiet revolution is in progress to suffuse every aspect of the CT value chain with smart tools informed through regression models, deep neural networks (DNNs), and other spoils of data science. Leveraged by “big data” initiatives that aim to support the analysis of vast numbers of CT exams from diverse populations, these methods will better inform if, when, and how frequently CT should be performed in support of clinical decision making. From adrenal nodules to pancreatic cysts, splenic artery aneurysms, aortic root dilation and pulmonary nodules, scientific evidence is frustratingly sparse in terms of when and how to follow myriad subacute and chronic abnormalities across a diversity of patient characteristics and pre-conditions.
Importance of Protocols
Next along the value chain lies the selection of optimized protocols and scan parameters. As CT technology advances and new clinical applications are developed, radiology departments often struggle to keep protocols up-to-date and sufficiently patient-specific. Anticipated to grow in their breadth and scope, recently introduced ML-informed scan parameter selection attuned to patient size and position dramatically simplifies the task of parameter selection and forms a basis for greater scan-to-scan consistency and diagnostic reliability.
With the scan completed, DNNs are poised to replace traditional reconstruction as the basis for constructing images from raw sinograms. While a reduction in image noise and artifacts will be a primary benefit, DNNs will provide a basis for converting images acquired with different CT systems to simulate uniform image characteristics, reducing the influence of scanner-based variability on feature extraction and characterization in radiomic applications. Combined with DNN-informed image segmentation, context-sensitive reconstruction strategies have been demonstrated to optimize noise, spatial resolution and display windowing across the diverse tissues and body regions (e.g., shoulders, hips, metal implant) encountered within the imaging volume. Finally, it can be expected that energy discriminating reconstructions will be optimized similarly.
The next handoff along the value chain will be to image interpretation. The scope of opportunity to automate and improve lesion detection and characterization is immense. It also offers the potential both to improve consistency of CT interpretation and quantification across readers and to extract clinically impactful information that is currently unassessed and potentially unrecognizable by human observers. Examples include quantification of bone demineralization, detection of intracranial hemorrhage, and early manifestations of diffuse lung disease.
Finally, with the CT data acquired and interpreted, analytical models will emerge that assess imaging results together with other patient data and clinical outcomes to enhance prognostication and management decisions.
In sum, we stand at the cusp of a number of transformative CT innovations that we can anticipate will bring fundamental changes to the technical capabilities and clinical applications of this diagnostic workhorse. With so many exciting possibilities, my hope for CT is perpetual youth.
Emerging CT Focus of RSNA Online Course
RSNA’s Online Learning Center offers the case, Photon-counting Detector CT: System Design and Clinical Applications of an Emerging Technology: May-June 2019.
Access this and all online courses at RSNA.org/Education.