Shining a Light on Photoacoustic Imaging
Powerful, noninvasive imaging tool holds promise for cancer, stroke
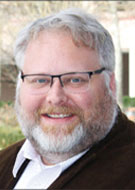
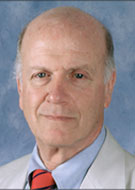
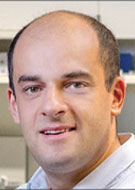
It is safe to assume that when Alexander Graham Bell discovered what is now known as the photoacoustic effect in 1880, he had no idea that it would become the basis for a groundbreaking new biomedical imaging technique more than 100 years later.
According to Randy King, PhD, program director, Ultrasound, Division of Applied Science and Technology at the National Institute of Biomedical Imaging and Bioengineering (NIBIB), the concept is fairly straight forward: A photoacoustic effect is caused by shooting light into tissue, which causes that tissue to heat at a microscopic level and vibrate, creating soundwaves that can be picked up with an ultrasound (US) receiver.
NIBIB continues to break ground in photoacoustic imaging research as part of the institute’s Optical Imaging and Spectroscopy Program.
“It’s a new, noninvasive, inexpensive imaging modality,” Dr. King said. “For a long time, photoacoustic imaging was more of a curiosity, but recently, with the application of specific molecules and contrast agents, it is emerging as a high-resolution, highly specific imaging modality.”
Imaging the Body’s Light Absorbers
The development of lasers capable of generating short, but powerful, bursts of light at different wavelengths was key to making photoacoustic imaging a viable imaging tool, said Reuben Mezrich, MD, PhD, chairman emeritus of radiology at University of Maryland School of Medicine.
As he explained in an editorial in the April issue of Radiology, these bursts of light create soundwaves in the area of the body where the light is absorbed. And by matching the optical wavelength to materials within the body, or those introduced into the body, such as contrast agents, it becomes possible to image various light absorbers.
For example, within the body there are natural absorbers of infrared light — of which hemoglobin is the most effective, Dr. Mezrich said.
“And hemoglobin is neat because the wavelength at which it absorbs light changes depending on whether it is oxygenated or deoxygenated,” he said. “By shining a light of one infrared color wavelength that corresponds to hemoglobin when oxygenated, we can detect oxyhemoglobin. If we shine a light that corresponds to oxyhemoglobin, we can get a picture of all the areas where oxygenated blood is perfusing. We see the blood vessels and arteries, and we will see tissue right next to capillaries. And we can also measure how much profusion is in a lesion."
Implications for Cancer, Stroke Diagnosis
Another potential application was discussed in a 2019 Radiology study by Ivana Ivankovic, PhD, from the Institute for Biological and Medical Imaging, Helmholtz Center Munich, Neuherberg, Germany, and colleagues, in which the authors were able to visualize the human carotid artery by using handheld volumetric multispectral optoacoustic (photoacoustic) tomography (MSOT).
“Our new MSOT technology has been shown to provide striking level of anatomical, functional and molecular detail, deep from living tissues and with high spatial and temporal resolution,” said study author Daniel Razansky, PhD, director of the Functional and Molecular Imaging Lab at the University of Zurich and the Swiss Federal Institute of Technology in Zurich.
Dr. Razansky co-authored an article on the history of photoacoustics. Several efforts are underway to apply it to non-invasive detection of breast malignancies, lymph node metastases, diagnostics and staging of inflammation and cardiovascular diseases, characterization of skin lesions, to name a few examples.
Potential Use in the Clinic
Adding photoacoustic technology to the clinic would not be complicated, Dr. King said. “It would be easy to implement. You basically need a light source — laser — and an ultrasound transducer to receive the signal,” he said.
However, the technology does have limitations, the most obvious being that light won’t penetrate tissue very deeply. “For example, noninvasive brain imaging would be very limited in terms of depth,” Dr. King said.
To achieve the desired specificity and resolution from photoacoustic imaging, a third component is needed in addition to the light source and the US transducer, Dr. King said. “A probe marker, a molecule, or a combination of molecules is needed. And you would need to optimize the photoacoustic effect, intensity, dose, toxicity — other components that come with injecting a contrast biomarker photoacoustic agent. That will add to the complexity.”
For More Information
Access the Radiology editorial, “The Potential of Photoacoustic Techniques for Molecular Imaging,” and the Radiology study, “Real-time Volumetric Assessment of the Human Carotid Artery: Handheld Multispectral Optoacoustic Tomography.”
Access the review, “Photoacoustics: a historical review,” in Advances in Optics and Photonics at osapublishing.org