Clinical 3D Printing: Status, Challenges and Opportunities
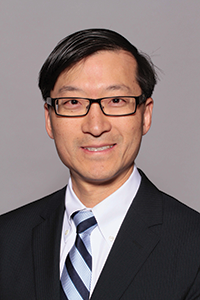
During the past several years, 3D printing has become increasingly common in the clinical setting, with application to a wide range of clinical challenges. In many areas, radiologists, imaging scientists and other imaging professionals have been the driving force behind these clinical advancements. As this technology continues to transition into the clinical workflow, there will be many challenges and opportunities ahead. Through collaborations with other specialties on 3D printing applications, radiology departments and practices can deepen their engagement with other clinical services, raise the utility of imaging to referring providers and improve the care of patients.
History and Current Status
3D printing was invented in the 1980s, and found its first uses in engineering and manufacturing. Clinical applications were not far behind, and the first descriptions of 3D printed patient-specific models derived from radiologic images appeared in the 1990s. Since then, parallel advancements in imaging capabilities and 3D printing technologies have enabled a proliferation of clinical 3D printing applications.
Some of the first clinical applications of 3D printing were in craniomaxillofacial surgery, and this remains an important area for clinical 3D printing. Today, printed models are also used in many other domains such as orthopaedics, vascular surgery, cardiology and cardiothoracic surgery, transplant surgery, urology and neurosurgery. These models may be used for surgical planning (e.g., selection of a surgical approach or selection of surgical devices) and surgical simulation (i.e., the use of models to practice surgical techniques or test deployment of devices prior to performing an operation).
Several other perioperative and intraoperative applications have also been developed, including the use of printed models to enable preoperative bending of fixation plates to fit a specific patient’s bony anatomy (thereby reducing operative time), as well as the creation of patient-specific cutting guides and surgical tools (to increase the precision of surgical maneuvers). In addition, 3D printing has been used to create customized, patient-specific implants and prosthe-ses. 3D-printed models have also been used extensively for medical training and education. For example, trainees in spine surgery have reported benefits from learning pedicle screw placement with printed models. Printed models are also used to communicate with patients about diagnoses and procedures.
The patient-specific nature of these 3D printed models and devices derives from the use of cross-sectional imaging as the source of an individual patient’s anatomy. Furthermore, the construction of these models involves image interpretation and clinical judgment, such as with regard to lesion characteristics, the relevance of particular image findings and the assessment of image noise and artifacts. On the other hand, the specific clinical challenges which make printed models so useful are often most familiar to surgeons and other referring colleagues. Consequently, imaging departments and radiology practices are well-placed to serve a central but collaborative role in the development of 3D printing capabilities within a health care enterprise.
A typical workflow for creating image-based 3D printed models starts with isotropic or near-isotropic CT or MR images. Image filtering may be applied to reduce image noise. Target structures are identified in the images through the process of image segmentation. Segmented structures are then used to create surface models, where 3D objects are described by triangulated surfaces. Post-processing allows for operations such as surface smoothing, gap filling and error correction. Additional modeling at this stage enables the introduction of other features, such as a minimum wall thickness for hollow vascular models, cut planes to show internal structure, or engineered features such as drill holes through a surgical guide. Surface models are checked against the source images for fidelity, and these models may then be used as input to a 3D printer. Depending on model complexity and the type of printer used, various post-processing steps are typically required after printing is complete, such as the removal of sup¬port structures, cleaning of extraneous substrate material or the curing of printed models. Finally, printed models are checked for dimensional accuracy.
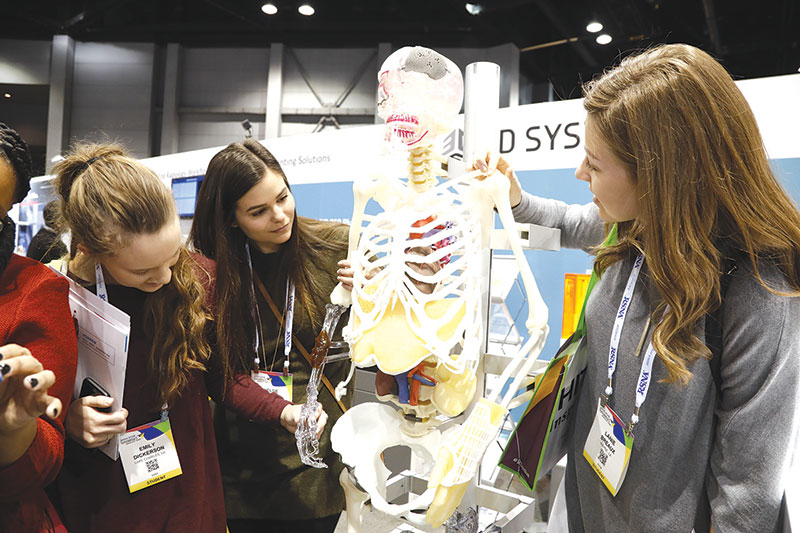
Trends and Challenges
Several evolving trends, issues and challenges will shape the nature of clinical 3D printing in the years ahead. These fall into a few broad categories: technical, commercial, regulatory and administrative.
Technical advancements in 3D printing technologies are expected to continue along several fronts. The first of these will be materials and printers. There is an ongoing demand for ever more physiologic-like 3D-printed materials at lower price points. The available options, such as soft, flexible materials that mimic biologic tissues, continue to improve in terms of physical properties and cost. In addition, whereas traditional 3D printers could typically print either a single type of material for a given model, or perhaps a range of material types in discrete regions, newer printer technologies now permit more continuous variation in printed mate¬rial properties.
Second is bioprinting, which refers to the use of 3D printing to create scaffolds for tissue growth, or the direct printing of cells or biomaterials. This technology will continue to advance, enabling the evolution of these techniques beyond the laboratory into clinical use.
The third area for growth is machine learning (ML). This subset of artificial intelligence has received a great deal of attention in recent years. In the realm of 3D printing, there is potential for ML-based techniques to dramatically reduce the time and effort required to create high-fidelity surface models from imaging data.
Evolving in tandem with these technical advancements will be changes in the commercial space for 3D printing technology. There are already a variety of ways to access these technologies, ranging from an entirely in-house approach, where a department develops a complete suite of internal capabilities in image segmentation, model construction and printing, to an entirely outsourced approach, where models are provided by vendors as a service, based on imaging exams. The pricing within this space also varies, reflecting the different types of software, materials and printers. As new technologies emerge, and as clinical applications mature, the disposition of users and vendors to the business models of clinical 3D printing is likely to evolve.
Regulatory issues will also play an important role in the future evolution of clinical 3D printing. In the U.S., medical devices are subject to regulatory oversight by the Food and Drug Administration (FDA). The FDA is actively tracking the clinical 3D printing industry and has provided clearance for several software products to produce surface models from imaging data.
There is now at least one system that has received FDA clearance for a more complete workflow including software for segmentation and model construction, as well as specific machines for 3D printing of diagnostic models in specific anatomic locations. The regulatory landscape will continue to develop with the advent of new materials, surgical guides, printed implants, bioprinted devices and ML-based techniques.
Finally, the practice of clinical 3D printing faces a number of administrative challenges. The cre-ation of high-fidelity models can be time-intensive, although this work is currently not reimbursed by the Centers for Medicare and Medicaid Services (CMS). It falls to practice leaders to consider the allocation of staff time and other resources in this environment, while weighing the potential benefits of providing this service. Future reimbursement will depend on systematic collection of usage data, and demonstration of clinical impact. A recently approved category III Current Procedural Terminology (CPT) code for 3D printing will aid in these efforts. In the future, if an application for reimbursement is successful, questions about turf and ownership may become more acute.
Opportunities Ahead
Although 3D printing is not new, the clinical use of this technology is entering a dynamic phase characterized by a wide range of clinical applications, on-going technological advancements and increasing levels of adoption. As imaging experts and trusted data stewards, radiologists and imaging departments are well-positioned to continue leading efforts in this arena. In doing so, they have the opportunity to engage with other services around key clinical challenges, to make imaging even more useful to referring providers, and to drive quality improvements in patient care.
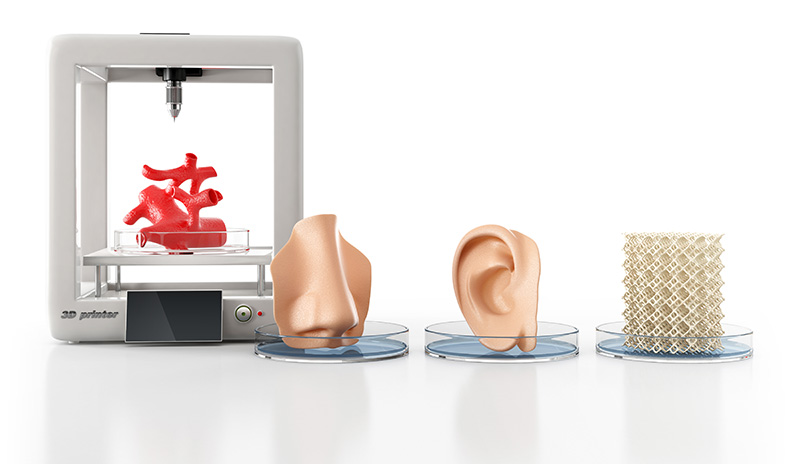